INTRODUCTION
Coastal areas like Malibu are subject to some degree of hazard due to ocean waves. Damaging ocean waves can be generated by high winds or changes in the sea bottom configuration that sometimes accompany an earthquake. Those due to strong winds are generally referred to as "storm waves;" those due to displacements of the ocean bottom are referred to as "tsunamis," the Japanese word for "harbor wave." In Malibu, as elsewhere, the risks of the storm-wave hazard and the tsunami hazard are distinctly different, the latter generally much more serious. However, an elaboration of the character and risk of storm waves is helpful in clarifying those of tsunamis.
Ocean waves - and in fact any waves in a liquid medium the surface of which is open to less dense medium such as the atmosphere - are manifestation of a system in which energy is being mechanically transmitted. They are sometimes referred to as "gravity waves" because once energized, gravity forces them to return to the level of static equilibrium. Such waves - whether generated in a laboratory tank or the open ocean - indicate that what are perhaps best described as "energy pulses" are being transmitted as a result a force being applied in such a manner that it invests, at any given instant, energy in a discrete mass of water that then propagates in a specific direction. It is the energy that propagates - not the water - and it does so as compression waves much as do sound waves through the atmosphere and seismic P waves through the earth. Within the energized water mass, the energy is distributed in near-accordance with the principles of the conservation of mass, momentum, and energy - near because a small amount of the energy is lost as frictional heat. The controlling physical characteristics of the system are the mass of the energized water at any given moment, its virtual incompressibility, its lack of strength, its homogeneity, and - at inception at least - the tensile strength of its surface.
STORM-WAVE HAZARD
Historically in Malibu, the risk of damage from storm waves has been quite low. Nevertheless, it should be considered, because the character of such damage can be very serious and, in fact, fatal. Therefore, the basic characteristics of wind waves, the manner in which they are transformed upon meeting shorelines, and their potential for damage onshore are addressed here.
Ocean Wave Formation
Generally, the study of water waves is approached by the use of mathematical models that approximate how mechanical energy it transmitted though it based on the manner in which the surface is deformed during such passage. The models that seem closest to real conditions are those of the trochoid wave in deep water, which like the sine function is - for want of a better term- axially symmetrical, and the cnoid wave in shallow water which is not.
Such models are used to calculate wave length and wave amplitude, The depiction in texts of a water wave as a sine wave, although convenient for purposes of description, does not generally represent the form of real water waves. As normally presented in the literature - the typical sine-wave diagram purports to show circular orbital paths of water particles. It is to be understood, however, that as the energy passes, the entire energized water mass as a unit undergoes an orbital motion. In other words, every point or volume within the energized mass has orbital movement relative to its position in space. Further, as fully developed in the open sea where wave length is much greater than wave height - the orbital movement is elliptical. As a matter of surmise, it requires more energy to excite a water mass under greater pressure, and as a consequence the volume of energized water decreases with depth. Hence, orbital movements lower in the energized mass have diameters smaller than those higher in it.
Ocean waves begin by the transfer of wind energy to the ocean surface over a specific area called a fetch. Through a somewhat uncertain process probably as a consequence the surface's tensile strength, ripples initially form. In theory, as the wind acts more forcefully on their windward sides than on their leeward sides, ripples begin to increase in size and length forming waves in which the continued addition of wind energy to the mass raising the water surface is counterbalanced by the force due to the gravitational acceleration acting on that energized mass. In terms of energy transfer, work by the wind the raises a mass of water above the local sea-level datum forming the wave crest thereby giving it potential energy. The mass then is then converted to kinetic energy as the crest descends to the wave trough in response to gravity. Therefore, whatever the amount of potential energy of the wave mass due to its position above sea level at any given instant must be the same as the amount of kinetic energy of the mass due to its descent below sea level at that instant. Put another way, the work of raising and lowering similar volumes of water must be equal. At all times therefore, there is a constant exchange of equal amounts of kinetic and potential energy in the energized water mass.
The result of wind working in the fetch is the formation of a discrete energized water mass within which individual waves travel with constant velocity, direction, and wave period. Although sea water is not exactly homogeneous because of variations in temperature and salinity, and the earth's gravitational acceleration is not the same everywhere, it is reasonable to assume the values of these parameters are essentially constant because the system retains its energy except for the minor loss of heat. Depending on the variations in wind force and direction, various such energized water masses eventually emerge from the fetch, each functioning as a group of waves. These groups represent pulses of energy propagating away in various directions depending on local generating conditions within the fetch. It seems as though, as a kind of entropic behavior, the length of the wave group would increase, and perhaps it does, but so slowly that it is not obvious. However such behavior would be difficult to observe, and I've yet to find any discussion of it in the literature.
At any rate, since wave groups emerging from the fetch have different velocities, they become more and more separated the farther they travel. In the approximation of wave behavior referred to as "Airy wave theory," it is demonstrated that individual waves within the group travel at a velocity, called the "celerity" or "phase velocity," that is twice that of the group itself. Consequently, in a process many find non-intuitive, this train of individual waves within the group seemingly are continually decaying at the advancing edge of the group and being generated at the trailing edge. This fascinating behavior of movement within movement, as it occurs in a laboratory tank, is described by Bascom (1980, pp. 71-72).
When ocean waves of a group have become well formed, they become "swell." Higher swell that is especially well formed is indicative of a distant and especially active fetch. In surfing terminology, a group of swell approaching the shore is called a "set." As a matter of observation, it is common, in Malibu at least, for as many as ten or more well formed sets, to reach the shore on a good surf day. From experience, the period between waves within a set can be two or three minutes, and the period between sets can be ten to twenty minutes.
Mechanics of Shoaling Wind Waves
Waves of a set moving into shoal waters take on different shapes depending on local conditions - most significantly, those of water depth and bottom configuration. In this process, the shoaling wave presents an observable change from one energy state to another. Except in the special circumstance where the shore is simply a steep cliff, the approaching is beginning to "feel the bottom" which means that the bottom is beginning to interfere with the wave's orbital movement. Then, all sorts of stuff starts to happen. Orbits lower in the energized mass are observed to adopt linearly alternating paths parallel to the bottom, and so far as my cursory examination of the literature discloses, no one yet has hypothesized on how this affects orbital movement higher in the wave. It's as though the total energy of the wave begins to "bunch up," to use handy if not particularly physics-OK imagery. This is because, theoretically, the total energy of the individual wave must be conserved in that wave, and because its period, which was developed during its formation in the fetch, is constant, regardless of the water depth. As explained by Airy wave theory, the velocity of individual waves within a group, referred to as the "celerity," is a function of the gravitational acceleration, g, and the ratio, d/L, of water depth, d, to the wave length, L. Furthermore, when d/L reduces to a value of less than 0.05, i.e., when the depth is less than one twentieth of the wavelength, the celerity, C, is approximated as the product of gravity and depth to the one-half power:
C = (gd)1/2 .
This means that as the water shoals toward the shore, the wave slows. For example, the celerity of an incoming wave with a d/L less than 0.05, at a depth of 15 feet would be about 22 feet per second and at a depth of 10 feet would be about 18 feet per second. Furthermore, the celerity is at all times a function of the wave length and wave period, T:
C = L/T.
Here, celerity varies directly with the wave length, and the wave period is the constant of proportionality. Therefore, since the farther inshore a shoaling wave advances the less its d/L value, the less its celerity, and the shorter its length. These simple relationships describe the manner in which waves meet the shore, and that in turn sets the stage to consider the storm-wave hazard. For those whose intuition is bothered by the fact that the period is constant, reference is directed to Eagleson and Dean (1966, pp. 21-24).
Changes in Shoaling Wave Form
As a working hypothesis, conservation of energy requires the incoming wave height to increase as the wave length decreases. A wave approaching shore at a particular point may develop a height and a steepness sufficient to be surfable. Clearly, as the height of a wave moving inshore begins to increase, so does its potential energy, and by hypothesis this must be at the expense of its kinetic energy which in the case of a shoaling wave presumably must be partly that of orbital movement and partly that of alternating flow trajectories along the bottom. Except for dropping in from the curl - from a standing start so to speak - catching a wave on a board requires sufficient velocity, reached by paddling, to begin sliding down the wave front. In that process, one feels a definite forward push. This is a result of the advancing and steepening wave front that causes the normally vertical component of the buoyant force to tilt shoreward, hence resulting in a lateral component in that direction which, helped by one's own effort, imparts an acceleration, and away you go, cutting right or left to avoid the break.
As an aside to the uninitiated, the break of a surfable wave progresses laterally along its crest allowing one to keep ahead of it, and by fancy maneuvering on the unbroken, steeper wave face, demonstrate what a fine fellow one is. Cutting right or left from the break is a very good idea. Simply taking off and remaining in the direction of wave propagation as the wave breaks is seldom any fun, especially at low tide. Interestingly, a similar action can be experienced, for example, in rowing a dory or piloting a small boat at sea in the same direction as swell. Matching and then slightly increasing velocity over that of the swell presents a slope down which to slide much like a surfing take-off, but unlike surfing, maintaining the direction of the wave rather than cutting across it. This technique is called, locally, "railroading," a term that may have been derived from the free rides the railroads provided hobos during the depression days following the '29 stock market crash. In fact, surfing along the southern California coast seems to have begun in the early 1930s as a form of recreation by young men who had little or no employment in those days. But that's another story.
The constant exchange of wave height potential energy and kinetic orbital energy in the open sea is replaced in shoaling water by the kinetic energy of flow over the shore. Approaching waves differ in length, height, and period, depending on the manner of their generation in the fetch, and further, they differ in their manner of development and form inshore depending on the gradient of the shoaling bottom. Generally, a wave of the same length, height, and period, will break as a plunging breaker, or a spilling breaker, or not at all, depending on the bottom configuration, but in any case, it is obvious that force-wise - excuse the expression - the effects can differ dramatically at the shore. The air-entrained water of the broken wave, the "soup" to use a surfing term, has lost most of its potential energy and a third kind of energy - that of kinetic flow - is introduced at the expense of the former two. Furthermore, this conversion to more or less well defined flow is not confined to the breaking wave. Rather, a kind of flow perhaps best regarded as incipient, occurs in the mass of a surging wave.
Investigation in this regard has been relegated for the most part to laboratory experiments. Figure 1, for example, illustrates the results of one in which the paths of neutral-density marker drops in a wave-model tank are plotted as the wave starts to break. It is apparent that the orbital movement of water waves in the open sea has been largely modified to one of lateral translation resulting from the effect of a shallow sloping bottom.
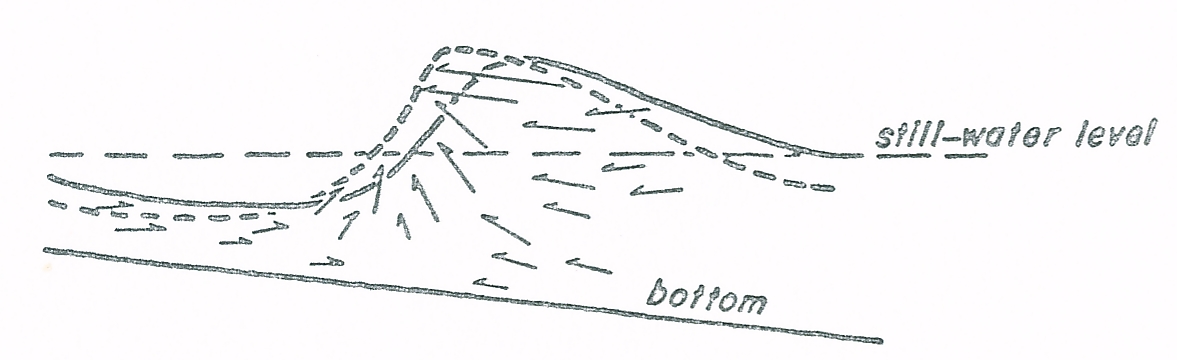 |
Figure 1. Water Particle Movement in a Model Wave Tank
This illustration from Bascom (1980, p 38) shows directions of neutral density "goop" drops as captured on film. They represent movements of individual water particles. |
Arrows in the figure show vectorial trajectories of the water. Those pointing right, seaward, in the wave trough represent movements of drops following passage of a previous wave. Those in the body of the wave pointing left, shoreward, represent movements of drops in the cresting wave. The orientations of these vectors demonstrate how orbital movement degenerates into a sort of incipient flow movement of a surging wave that may pass on to the shore without actually breaking.
The surging wave expends its energy as flow without breaking in either of two situations. One is where it meets a shore bottom that is too steep to allow time for either a plunging or spilling configuration to develop as when a wave encounters reef or a submarine cliff. The other is where the bottom gradient is very low and the ratio of wave height to wave length is too small for a significant height to develop. Such conditions occur in partially enclosed shore areas such as harbors or small embayments and they are similar to conditions that develop when such areas receive tide waves. A tide wave differs from an ocean wave in that it is not generated by wind. In fact, where the ocean wind waves pass over the earth, the earth passes under the tide wave.
Two rather dramatic instances of surging wave action due to tides are worth mentioning. One is that of the tide bore that develops in the mouths of some streams or in some bays, e.g., the Bay of Fundy in Nova Scotia. The other is the effect of normal tide surge such as that which developed when Marina del Rey, in Venice, California first opened. There, it seems that partly as a result of claptosis and partly surging tide flow, currents and waves damaging floating slip facilities and boats in them commonly occurred. Whether the designers simply did not foresee such behavior, or initially decided because of economics to wait to see the tidal effect, is undetermined or at least not publicized. But in any case, the problem was not solved until a breakwater was constructed outside the harbor channel entrance. But I digress.
Malibu Storm-wave Hazard
Storm waves great enough to be hazardous in Malibu are those generated by the especially strong and persistent winds of low-pressure tropical cyclones that develop seasonally in the southeastern Pacific north of the equator. A single reported occurrence of a cyclonic winds that came ashore in the Long Beach area in 1939 caused considerable flooding and wind damage. However, the largest swells the southern California coast receives are from such cyclonic disturbances. Associated storm waves, even those generated by far distant winds, can be especially destructive in certain circumstances. Furthermore, although there is some reason to believe that waves of a given storm are more hazardous to certain stretches of coast than others because of bottom focusing, the available data are insufficient to conform this.
The original Santa Monica Pier - one constructed of driven timber pilings - was lost in this way. I believe it was on March 1, 1983. I was on the pier that day, and the swell was unusually high. It caused a single timber whaler, unused for many years but still tied at the end of the pier, to break loose. As I recall, it was about 18 inches square and perhaps 20 feet long. Incoming waves began using it as a battering ram, and this soon caused several piling bents to become dislodged. As a result, individual pilings started to break loose because they were seated perhaps only a foot or so into the bottom bedrock which there I believe is section of sandstones and siltstones (probably Monterey Formation for all you stratigraphers). Rather, they had been installed simply by driving them to refusal - a practice locally common during much of the 20th Century. With the loss of bracing and additional pilings acting as battering rams, the horizontal strength of the pier was compromised. This weakness spread until - within about an hour, as I recall - much of the seaward end of the pier had collapsed. As to the relevance of this - many of the houses on Pacific Coast Highway east of Las Flores Canyon and many along Malibu Road are built on driven timber pilings which extend at most a few feet into bedrock.
Storm waves coupled with higher high tides and storm surge has, over the years, resulted in localized damage to a few beach-front properties in Malibu. In some sixty years in Malibu, I know of only three instances of serious storm-wave damage, although there may have been a few others. One affected some houses along Pacific Coast Highway east of Las Flores Canyon, and another was a reported instance of waves breaking through windows of the Sea Lion Cafe - now called Dukes. In neither was there any reported injury that I recall. However, in a tragic incident on Malibu Road many years ago, especially high storm waves caused the pile-supported terrace of a residence to collapse. This fatally injured the owner, a friend I had known since high school days. He had been observing the waves from it at the time.
One way to lessen the risk of the storm-wave hazard is through better design of coastal structures; another is to deal with it circumspectly in terms of proximity. Much the same can be said for the other wave-associated hazard to which Malibu is subject - the tsunami.
TSUNAMI HAZARD
Tsunamis are generated by forces associated with earthquakes - generally those acting in or near abyssal trenches where, as a result of sea-floor spreading, oceanic tectonic plates are plunging below those of continents. However, whether the generation of a tsunami occurs only by a permanent volumetric displacement of the sea bottom or simply by a temporary transient change in the bottom configuration upon receiving seismic waves from a distance locus is uncertain. Furthermore, although some authorities reserve the name "tsunami" to waves generated by permanent earthquake-related sea bottom-displacement, others also refer to bottom-displacement waves generated simply by landslides of either terrestrial or marine origin unrelated to earthquakes as tsunamis. In any event, except as to scale, much of that is discussed concerning the behavior of storm waves also applies to tsunamis.
Tsunamis of two different origins may be hazardous for Malibu. One is that having a distant source - such as happened at Crescent City in northern California during the March 27, 1964 Cook Inlet, Alaska earthquake, and most recently during the March 10, 2011 Sendai, Japan earthquake. For tsunamis that travel great oceanic distances, the term "teletsunami" has come into use. The other is that which might be generated by movement along the Raymond Hill - San Miguel Island (RHSMI) fault zone a section of which lies immediately offshore from Malibu. Tsunami-generating seismic events from neither is predictable in terms of time of occurrence nor magnitude. Consequently, practical safety measures are limited to warnings of possible tsunami approach. In this regard, a recent Los Angeles County effort conceived as a tsunami safety measure through the use of road signs in Malibu intended to indicate escape routes is singularly wrong-headed and in some circumstances could result in actually dangerous conditions and even fatalities. The especially serious potential hazard of the tsunami justifies a brief departure here to better understand the tsunami mechanism.
Tsunami Mechanism
Whereas the energy of wind waves is transmitted to a large area of the water-atmosphere interface over a lengthy period, that of tsunamis arises from an almost instantaneous displacement of the sea bottom affecting a relatively small area. Once created, tsunami energy probably passes through the water in much the same way, mechanically, as does that of wind waves except as to scale. However, there seem to be no reports of tsunamis occurring in sets as do wind waves. If that happens, either of two mechanisms is suggested. One would be an episode of periodic incremental sea-bottom displacements along one or more faults. The other, theoretically at least, could be displacements of the bottom due to the arrival of seismic waves that would impart P-waves to the water mass. This suggests the interesting idea that the resulting water-borne compressional waves, upon meeting the sea surface are constrained to deform it in a manner analogous to that of terrestrial Rayleigh waveformation, the primary distinction being that the terrestrial Rayleigh waves respond elastically whereas water waves respond gravitationally.
Teletsunamis have much greater lengths than those of wind-generated waves. In the open ocean they travel at much higher velocities but with amplitudes commonly undetectable except by instruments. Nevertheless, upon approaching the shore they change in a manner similar to that of wind waves, again, except as to scale. The reason tsunamis are especially hazardous is that their volumes are much greater than those of wind waves. However, the extent to which the tsunami becomes dangerous depends not only on the configuration of the shore bottom but also on that of the adjacent terrain including, obviously, the kind of local development. In the case of a breaking tsunami, the water advances essentially as "soup" flow which, although having some impact force, and causing relatively shallow inundation, is most damaging and life-threatening due to the entrainment of debris. In the case of surge, the primary damaging effect of the water is a result of the impact force of the water mass. It almost certainly was such a surging mass that carried away the Scotch Cap lighthouse on Unimak Island during the April 1, 1946 Aleutian earthquake. Similarly, it was a landslide-generated surging mass during an earthquake that swept away timber stands as high as 1,720 feet above the level of Lituya Bay in Alaska in 1958.
Teletsunami Risk to Malibu
The magnitude-9.0 Sendai earthquake of March 10, 2011, as catastrophic as it has been for Japan, also has proven, technically, to be advantageous for California generally and Malibu in particular. This is because it strongly suggests that the teletsunami risk is of relatively minor concern with regard to the California coast. Damage in California from the teletsunami generated during the Sendai event reportedly has been was limited to certain harbors on the northern coast where surging resulted in unusually strong currents that damaged floating installations much as happened at Marina del Rey due to tidal flows discussed above. In Malibu itself, which lacks any kind of enclosed coastal areas, artificial or natural, no damage occurred from the Sendai event. Probably, it did not affect the shore there in any way different from the normal daily coastal wave or tide regimes.
Nevertheless, although this suggests that teletsunamis generated from earthquakes of even the greatest magnitudes recorded do not present a significant risk to Malibu, it is to be understood that there are no data demonstrating that the character of a teletsunami is directly a function of earthquake magnitude. This relationship remains somewhat in doubt because the extent to which earthquake magnitude is related to sea bottom offset, or in fact any other tsunami-generating mechanism, is undetermined.
Local Tsunami Risk to Malibu
A tsunami generated from a sudden, seismic offset along the RHSMI zone is possible. Although intense studies have a provided a statistical basis for predicting a significant seismic event along the San Andreas fault - in the next 30 years, I believe - there are no such data for faults the of the RHSMI zone nor elsewhere in California so far as I know. Clearly, the RHSMI zone is active, but nevertheless, the occurrence, location, magnitude, and duration of earthquakes along it are not predictable. Were an earthquake accompanied by a fault offset along an oceanic section of the RHSMI zone to occur, the initial movement probably would raise the sea bottom landward of it causing a tsunami, and since the zone is nearby, very little time would elapse before it reached shore. The following elaborates on an earlier discussion regarding certain aspects of Malibu's tsunami risk.
As an intellectual exercise, and only that, assume a fault offset in the RHSMI zone two miles offshore from Malibu. Further, assume that the effective bottom depth from the fault for a distance of a mile inshore is 60 feet and from there to the shoreline 40 feet. Finally, assume one can apply Airy theory with regarding to a shoaling wave generated locally - which is not at all certain - a tsunami should have, initially, a velocity of about 44 feet per second, or about 30 miles per hour. Farther inshore, it would slow to about 35 feet per second or about 24 miles per hour. So the tsunami would take about 2 minutes to travel from the point of initiation through the mile-wade 60-foot depth area and then about 2.5 minutes through the remaining mile from there to shore. Consequently, less than about 5 minutes would be available to get to ground high enough to be safe, depending on the tsunami height. It is relevant to note that a tsunami, like any water wave in fact, is always heralded by an initial withdrawal of the water, except that in the case of the tsunami the level falls well below the normal trough elevation prior to the crest reaching the shore (see. Figure 1). One must judge for oneself, particularly in terms of location, what this means in terms of safety. What it almost certainly doesn't mean is that there would be enough time to grab the kids, jump in the Ferrari, and race to one of the County-recommended tsunami evacuation routes. That is why such "signage" - horrible term that - is so wrong-headed.
Another example of wrong-headedness, not so serious but still wrong, is an idea circulated some years ago by the County, that a landslide from a submarine slope - presumably in Redondo submarine canyon off the northwestern coast of Palos Verdes Peninsula - could generate a tsunami causing an uprush of "40 feet" onshore in Malibu. As apparently is the case for any submarine landslides that have occurred in the historic past in Santa Monica Bay, the resulting water-volume redistribution has not been sufficient to generate a tsunami. This could be because any such slide mass that may have occurred would have been too small, or move too slowly, to develop the requisite bottom change. Furthermore, any such effect would have been confined to some extent by claptosis from the opposite canyon slope. Equally important however, a submarine landslide, as an essentially point-source disturbance, would result in a seismic sea-wave approaching Malibu with crests as arcuate segments. Since:
[i] water-wave energy varies directly with wave height,
[ii] wave height decreases with increase in wave-crest length,
[iii] the wave-crest length from Palos Verdes would increase toward Malibu,
the heights of such waves upon reaching Malibu shores would be significantly decreased. Moreover, the very idea that everywhere in Malibu uprush would be the same, i.e., 40 feet or whatever, is patent nonsense, not only because there is no apparent basis upon which to postulate the size of a landside that would cause an uprush of any dimension, but because of attenuation of the wave height with distance away from the source. West Malibu is farther than east Malibu from Palos Verdes Peninsula. Capisce? An inquiry I made to the County at the time regarding how the 40-foot uprush was calculated was ignored. No surprise there.
CONCLUSIONS
Risks presented by ocean waves in Malibu are, if not minimal, certainly not especially serious based on the historic record to date. Certain existing structures along the shore should be evaluated in terms of wave damage risks and in some cases improved structurally based on professional recommendations. Most critical - if that is the word - is the risk of a locally generated tsunami. Two - no, make that three - remedial approaches are obviously suggested. The first would be to introduce a siren warning system - something like that reported to operate in Hawaii. The second would be the development of specific plans for pedestrian escape routes - perhaps in some way tied by a City ordinance, like that requiring a septic-system inspection as a condition of property title transfer - which of course would differ for different coastal localities. And the third would be to get rid of all those ill-advised tsunami evacuation route signs which are not only useless, but can be dangerously misleading.
* * *